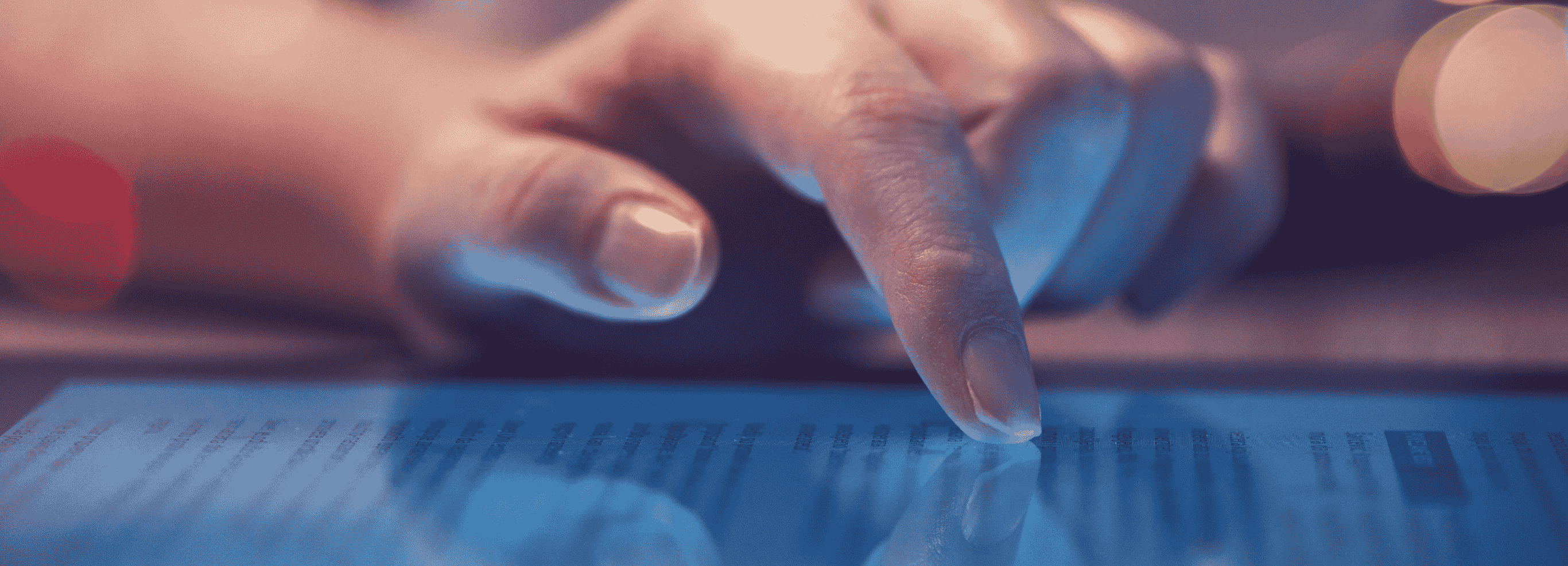
Vaporized Hydrogen Peroxide: A Well-Known Technology with a New Application
Vaporized Hydrogen Peroxide: A Well-Known Technology with a New Application
Brian J. McEvoy B.Sc. MBA1, Randal Eveland Ph.D2 1 Senior Director Global Technologies, STERIS, Tullamore, Ireland. 2 Senior Manager Research & Development, STERIS, Mentor, Ohio, USA.
Abstract
Hydrogen peroxide has a multitude of uses and the vapor form was first identified as a sterilant in late 1970s. Following a number of developments, vaporized hydrogen peroxide (VHP) became widely adopted in early 90s as a substitute for ethylene oxide (EO) in device and instrument processing and reprocessing in healthcare facilities. Often VHP was hailed as the replacement technology for EO. Because of key limitations such as scale, penetration, and compatibility with packaging materials, adoption to terminal sterilization of single-use devices has not commenced to any significant level. However, recent developments in sterilization chamber design and process development provide new opportunity for consideration. For future products, such as those that require “end of production line sterilization,” such limitations may be reconsidered and overcome. This article describes those challenges and how they have been addressed, with practical examples. The development of global consensus standards and leveraging the well-established knowledge of VHP sterilization with regard to microorganism inactivation and material compatibility will help facilitate wider consideration of VHP technology as a true alternative to EO in certain product applications.
Introduction—Terminal Sterilization of Medical Devices
Terminal sterilization of single-use medical devices may be broadly subdivided into two main technologies, namely radiation and sterilization by ethylene oxide (EO) gas.[1] The continued reliance on EO is a consequence of its wide-ranging material compatibility and availability for scale-up. The challenges of using EO relate to the hazardous and carcinogenic nature of the gas coupled with the prolonged treatment times. Radiation, on the other hand, is a relatively quick process with no toxic residues, but is limited by the availability of the radiation source (cobalt, in the case of gamma irradiation) and material compatibility, described in AAMI TIR17.[2] Therefore, a continual need for extension of the available terminal sterilization technologies remains and was highlighted in 2019 with the launch of the Food and Drug Administration (FDA) Innovation Challenge to identify new sterilization methods and technologies. Given its extensive history in healthcare processing and being deemed a Category B sterilization method by FDA, vaporized hydrogen peroxide (VHP) is now being considered as such a candidate technology for inclusion.
Vaporized Hydrogen Peroxide
With hydrogen peroxide established as a biocidal agent since the 1800s and the first uses of the vapor form in sterilization emanating from the late 1970s, VHP is a well-established sterilization method of choice in the healthcare setting for the processing of reusable devices.[3] Furthermore, VHP is used in many other applications, such as room and facility decontamination.[4] The disinfection and sterilization efficacy of hydrogen peroxide in both aqueous and gas form are well documented,4,[5] with the latter form considerably outperforming the liquid system.4,[6] It has been suggested that VHP can penetrate the three-dimensional protein structure and cause breaking of bonds between subunits more easily than liquid hydrogen peroxide.[7] The International Agency for Research on Cancer (IARC) has determined that hydrogen peroxide’s carcinogenicity to humans is not classifiable.[8] The advantages of using gaseous hydrogen peroxide are described by Hultman et al.6: 1) it will have uniform contact with all exposed surfaces, including those with complex topographies; 2) it may be safely maintained in a chamber environment; and 3) it may be efficiently and quickly removed from a chamber. Hydrogen peroxide is a strong oxidizing agent, having multiple targets within a cell as well as in almost every biomolecule: it can react strongly with thiol groups in enzymes and proteins, DNA, and the bacterial cell membrane.[9] Such a strong oxidizing agent can cause the formation of radicals such as ferryl radical, which is formed from DNA-associated iron and has an important role in DNA oxidation.9 As highlighted by the work of researchers such as Young and Setlow,[10] Fichet et al.,[11] and Setlow,[12] the site of microbial inactivation by VHP appears to reside at the inner membrane.
Industrial Scale VHP
Industrial VHP sterilization chambers (Figure 1) [NOTE: Figures courtesy of STERIS] are typically one-half to four pallets (35–280 ft3). This is in contrast with large EO chambers, often ranging from 1,000 ft3 to 2,200 ft3. A distinct advantage with VHP sterilizers, however, is the minimal requirement for supplies and services: water, compressed air, electricity, and sterilant. This allows equipment to be supplied on a single skid and installed with ease, which may be advantageous for ‘in-line’ applications. Equipment may be commissioned and validated in a manner similar to a steam or EO sterilizer.
Limitations of VHP and the Role for Process Design
As VHP offers a number of distinct advantages, including material compatibility (as highlighted in AAMI TIR17 and Table 1 of this document), efficacious microbial inactivation, and lack of toxic residues, it does present some limitations that must be considered and addressed, namely: 1) material compatibility; 2) packaging; 3) equipment scale and throughput capability; and 4) surface penetration. Strategies to address such limitations may be summarized as follows.
Material Compatibility
VHP has a wide range of material compatibility but is limited whereby a small subset of materials must be excluded due to absorption and decomposition of the vapor. Liquids, powders, and cellulose materials are not compatible with the process.5Packaging
Packaging for VHP sterilization needs to be compatible with the sterilant and
allow for diffusion of the sterilant to the medical device. Packaging
materials that prevent VHP from reaching the devices (e.g., foil pouches)
should not be used; however, the external surfaces of the material such as
glass (e.g., a liquid-containing ampoule) will be sterilized. Nonwoven
polyethylene and polypropylene materials have been proposed by the Sterile
Barrier Association. Such materials provide a sterile barrier for the product
while allowing sterilant gases and steam to penetrate and escape quickly.
Corveleyn et al.[13] have
shown that H2O2 penetration across Tyvek package was
considerably greater (87.7% of reference concentration as measured inside the
package) compared to that of medical paper (30%). Therefore, it is essential
that devices are packed in a suitable sterile barrier. Cellulose is associated
with product packaging (cardboard) and instructions for use (paper), rather
than with the medical device itself. Cellulose can absorb H2O2
to such an extent that it reduces the concentration of H2O2
in the vapor phase, causing the cycle to abort. Another potential approach
might be lacquering or coating of absorbent materials like cardboard or paper.
Meszaros et al.[14] have
examined the impact of surface material on the process lethality, and did not
observe any deleterious effects with beechwood, although it is a
cellulose-based material. The authors have speculated the reason might be the
processed (lacquered) nature of the material. Also, as cardboard cartons must
be excluded, operators may incur additional handling of materials. Possible
mitigating strategies may involve the presentation of product in high-density
polyethylene tote box systems: Such totes have been used in the past in EO
processing to improve product residual outcomes by removing cardboard cartons
from the process. Figure 1: Picture of STERIS VHP® LTS-V
Equipment Scale and Throughput Capacity
As already described, VHP sterilizers lack large volume scale compared to EO sterilizers. However, VHP has the advantage of quicker and more efficient processing that goes some way to addressing the scale. Consequently, chamber size—coupled with additional handling requirements to exclude packaging materials—would render VHP most suitable for specific niche applications, typically at the end of production line applications. For deployment into contract sterilization offerings, supply chains must be designed to streamline product flow and handling.
Surface Penetration
There are many different VHP sterilization processes used in the various commercial sterilizers available today, but all these processes follow a similar three-phase pattern (Figure 2): preconditioning, sterilization, and aeration. [NOTE: Figures courtesy of STERIS] First, a deep vacuum is pulled to remove air and humidity from the load and to create an environment for injecting VHP to a maximum level while not reaching the saturation point at which condensation will occur. The importance of this step is highlighted in a study performed by Hultman et al.,6 where a drop in a maximum H2O2 concentration from 2,148 to 1,805 mg/L has been reported, as moisture content goes from 0% to 10%. In the second phase, usually a 35% liquid H2O2 (w/v; pH~3) is vaporized at over 100°C and the vapor is injected into the chamber in the form of pulses to reach a final concentration of 1–2mg/L. Following sterilant exposure, vacuum is applied and using either air or steam or a combination of both to remove sterilant. Process parameters—predominantly pressure, temperature, humidity, and exposure time—can be optimized to design high-performing cycles and ensure penetration to the desired surfaces for sterilization. In lumen devices, residual air may impede vapor penetration[15] and therefore cycle design should consider critical parameters including vacuum depth, sterilant concentration, and exposure time. While there was previously concern about hydrogen peroxide penetration and sterilization within long narrow lumens, stainless steel and flexible lumens (e.g., flexible endoscopes) are now sterilized by subatmospheric VHP systems in healthcare. Figure 2: Diagrammatic representation of a VHP cycle showing three phases of Preconditioning, VHP exposure and Postconditioning.
Validation of VHP Processes
Currently, there is no international standard that provides performance requirements for biological indicators for VHP sterilization processes. The process is therefore characterized in accordance with ANSI/AAMI/ISO 14937:2009/(R)2013,[16] which states that a microorganism of known high resistance can be used to demonstrate the microbial effectiveness of the sterilizing agent. In the U.S., the FDA regulates biological indicators used in healthcare facilities and has a set of testing requirements for the clearance of VHP biological indicators in the U.S. market. While FDA 510k regulations[17] require the use of G. stearothermophilus in the hospital setting as the most resistant organism, the 14937 standard—which is appropriate for medical device terminal sterilization applications—requires the consideration use of a biological indicator of known high resistance, similar to that as defined for EO sterilization in accordance with ANSI/AAMI/ISO 11135:2014,[18] where Bacillus atrophaeus is the reference microrganism. It is known that G. stearothermophilus is more resistant to vapor, whereas B. atrophaeus is more resistant when subjected to liquid hydrogen peroxide[19]. Selection of a biological indicator to challenge the process is an important step, as it consequently quantifies the microbicial inactivation of the process and therefore the process outcomes (sterility assurance and material effects). The validation process detailed in ISO 14937 is a familiar and accepted process, as it is very much aligned to that for EO gas sterilization18 where an overkill half-cycle approach is often adopted: Like EO validation, alternative approaches to validation are also available and may be appropriate if one is to consider a more targeted process with less overprocessing. EO processing solicits wide acceptance from industry as it is a known, trusted technology with a long history of use. Furthermore, by nature of having its own international consensus standard (ISO 11135) recognized by the FDA, it has Category A status. VHP has Category B status, given its long history in healthcare and available body of knowledge. In 2017, a new work item was proposed to the International Organization for Standardization for the creation of a process standard for VHP sterilization. This proposal was duly accepted and a working group (WG16) formed under Technical Committee 198 (responsible for sterilization). This committee has commenced work on the draft standard, ISO/CD 22441. Similarly, in Europe work is underway by CEN TC102 WG6 to develop an equipment standard (prEN 17180) similar to EN 1422 (applicable to EO sterilizers). Once complete, both standards would provide normative references and guidance for both equipment and the sterilization processes provided by such equipment in both healthcare and industrial settings.
Conclusions
Examination of current and past applications shows VHP sterilization to be an efficacious, material-friendly, and useful sterilization technology. Like with every sterilization technology, there are limitations (described in parentheses): steam (high temperature), EO (residues), E-beam (poor penetration), gamma/X-ray (material compatibility limitations). VHP too has limitations. However, such limitations are to be considered and innovations provided to increase the adoption of the technology. Expectations for future adoption as a technology for terminal sterilization of single-use medical devices may be founded on 1) compatability with a wide range of materials, including polymers, sensors and electronics, metals, and electrical components; 2) operating temperatures typically lower than EO processing; and 3) ready deployment of self-contained sterilization equipment. Therefore, temperature-sensitive products, sensor/electronic combination products, or those requiring sterilization at source of manufacture (e.g., 3D printed products) may be excellent candidates for such a sterilization solution. The proliferation of any technology in medical device sterilization is predisposed to its acceptance by those who require sterilization for their manufactured devices. With continued focus, investigation, and dissemination of knowledge and experience, the possibilities VHP adds to the technology portfolio available to manufacturers can be realized. As projects are completed with a diverse range of products, experience and knowledge are gained that may be shared in future publications.
Acknowledgements
The authors would like to thank and acknowledge the Kilmer Collaboration Modalities group for the cooperative efforts considering and exploring how additional sterilization modalities may be deployed and adopted by the healthcare products manufacturing industry, for the benefit of patient care. [dt_gap height="10" /][dt_divider style="thin" /][dt_gap height="10" /]
References
[1] Gamma Irradiation
Processing Alliance, International Irradiation Association. A Comparison of
Gamma, E-beam, X-ray and Ethylene Oxide Technologies for the Industrial
Sterilization of Medical Devices and Healthcare Products. 2017.
http://iiaglobal.com/wp-content/uploads/2018/01/White-Paper-Comparison-Gamma-Eb-Xray-and-EO-for-Sterilisation.pdf. Accessed June 2, 2020.
[2] AAMI TIR17:2017.
Compatibility of materials subject to sterilization. Arlington, VA:
Association for Advancement of Medical Instrumentation.
[3] McEvoy B, Rowan NJ.
Terminal sterilization of medical devices using vaporized hydrogen peroxide: a
review of current methods and emerging opportunities.
J Appl Microbiol. 2019;127(5):1403–20.
[4] McDonnell G. The Use of
Hydrogen Peroxide for Disinfection and Sterilization Applications.
In: Rappoport Z, Ed. PATAI'S Chemistry of Functional Groups. John
Wiley and Sons; 2014.
[5] Block SS. Peroxygen
Compounds. In: Block SS, Ed.
Disinfection, Sterilization and Preservation. Philadelphia: Lea & Febiger; 1991:167–81.
[6] Hultman C, Hill A,
McDonnell G. The physical chemistry of decontamination with gaseous hydrogen
peroxide. Pharmaceutical Engineering. 2007;27(1):22–32.
[7] Finnegan M, Linley E,
Denyer SP, McDonnell G, Simons C, Maillard JY. Mode of action of hydrogen
peroxide and other oxidizing agents: Differences between liquid and gas forms.
J Antimicrob Chemother. 2010;65(10):2108–15.
[8] IARC Working Group on
the Evaluation of Carcinogenic Risks to Humans, International Agency for
Research on Cancer.
Re-Evaluation of Some Organic Chemicals, Hydrazine and Hydrogen
Peroxide.
1999 World Health Organization, International Agency for Research on Cancer.
[9] Linley E, Denyer SP,
McDonnell G, Simons C, Maillard JY. Use of hydrogen peroxide as a biocide: New
consideration of its mechanisms of biocidal action.
J Antimicrob Chemother. 2012;67(7):1589–96.
[10] Young SB, Setlow P.
Mechanisms of killing of Bacillus subtilis spores by Decon and Oxone,
two general decontaminants for biological agents.
Journal of Applied Microbiology. 2004;96(2):289-301.
[11] Fichet G, Antloga K,
Comoy E, Deslys JP, McDonnell G. Prion inactivation using a new gaseous
hydrogen peroxide sterilisation process. J Hosp Infect.
2007;67:278–86.
[12] Setlow P. Summer
meeting 2013 - when the sleepers wake: The germination of spores of
Bacillus species. J Appl Microbiol. 2013;115(6):1251–68.
[13] Corveleyn S,
Vandenbossche GMR, Remon JP. Near-infrared (NIR) monitoring of H2O2 vapor
concentration during vapor hydrogen peroxide (VHP) sterilisation.
Pharm Res. 1997;14(3):294–98.
[14] Meszaros JE, Antloga
K, Justi C, Plesnicher C, McDonnell G. Area Fumigation with Hydrogen Peroxide
Vapor. Applied Biosafety. 2005;10(2):91–100.
[15] McDonnell G, Sheard D.
A Practical Guide to Decontamination in Healthcare. West Sussex: Wiley
Blackwell Publishers, John Wiley & Sons, 2012.
[16] ANSI/AAMI/ISO
14937:2009/(R)2013. Sterilization of health care products—General requirements for characterization of a sterilizing agent and the
development, validation and routine control of a sterilization process for
medical devices.
Arlington, VA: Association for the Advancement of Medical Instrumentation.
[17] Food and Drug
Administration. Biological Indicator (BI) Premarket Notification [510(k)]
Submissions. October 4, 2007. https://www.fda.gov/media/71134/download.
Accessed March 30, 2020.
[18] ANSI/AAMI/ISO
11135:2014.
Sterilization of health care products—Ethylene oxide—Requirements for
development, validation and routine control of a sterilization process for
medical devices.
Arlington, VA: Association for the Advancement of Medical Instrumentation.
[19] McDonnell G. Chemical
Sterilization. In: McDonnell G, Ed.
Antisepsis, Disinfection and Sterilization: Types, Action and
Resistance. Washington: ASM Press;
2007:191–216.